

An efficient and practical process for the synthesis of ramelteon 1, a sedative-hypnotic, is described. Highlights in this synthesis are the usage of acetonitrile as nucleophilic reagent to add to 4,5-dibromo-1,2,6,7-tetrahydro-8H-indeno[5,4-b]furan-8-one 2 and the subsequent hydrogenation which successfully implement four processes (debromination, dehydration, olefin reduction, and cyano reduction) into one step to produce the ethylamine compound 13where dibenzoyl-l-tartaric acid is selected both as an acid to form the salt in the end of hydrogenation and as the resolution agent. Then, target compound 1 is easily obtained from13 via propionylation. The overall yield in this novel and concise process is almost twice as much as those in the known routes, calculated on compound 2.
A Novel and Practical Synthesis of Ramelteon
State Key Lab of New Drug & Pharmaceutical Process, Shanghai Institute of Pharmaceutical Industry, State Institute of Pharmaceutical Industry, Shanghai 200437,China
Org. Process Res. Dev., Article ASAP
DOI: 10.1021/op500386g
Publication Date (Web): January 6, 2015
Copyright © 2015 American Chemical Society
*Telephone: +86 21 55514600. E-mail: zhouweicheng58@163.com.

Preparation of (S)-N-[2-(1,6,7,8-Tetrahydro-2H-indeno[5,4-b] furan-8-yl)ethyl]propionamide(1).
GAVE
white solid of 1(1.570 g, 85% yield, 99.8% ee). Purity by HPLC 99.6%.
Mp: 115−116 °C(113−115°C in literature 1 ).

1 H−NMR(400 MHz, CDCl3):
δ 1.39 (t, 3H); 1.63 (m, 1H); 1.83 (m, 1H); 2.02 (m, 1H); 2.16 (dd, J=8, 2H); 2.28 (m, 1H); 2.78 (m, 1H); 2.83 (m, 1H); 3.14 (m, 1H); 3.22 (m, 2H); 3.33 (m, 2H); 4.54 (m, 2H); 5.38 (br s, 1H); 6.61 (d, J=8, 1H); 6.97 (d, J=8, 1H).

13C−NMR(100 MHz, CDCl3):
δ 173.85, 159.56, 143.26, 135.92, 123.52, 122.28, 107.56, 71.26, 42.37, 38.17, 33.66, 31.88, 30.82, 29.86, 28.73, 10.01.
MS (ES+): m/z 282(M+Na) + .
[α]D −57.3(c=1.0, CHCl3, −57.8 in literature 1 ).
Anal. (C16H21NO2) Calc: C, 74.10; H, 8.16; N, 5.40; found: 74.09; H, 8.17; N, 5.47.
References
(1) Uchikawa, O.; Fukatsu, K.; Tokunoh, R.; Kawada, M.; Matsumoto, K.; Imai, Y.; Hinuma, S.; Kato, K.; Nishikawa, H.; Hirai, K.; Miyamoto M.; Ohkawa, S. J. Med. Chem. 2002, 45, 4222-4239.
(2) Yamano, T.; Yamashita, M.; Adachi, M.; Tanaka, M.; Matsumoto, K.; Kawada, M.; Uchikawa, O.; Fukatsu, K.; Ohkawa, S. Tetrahedron: Asymmetry. 2006, 17, 184-190.


SHANGHAI
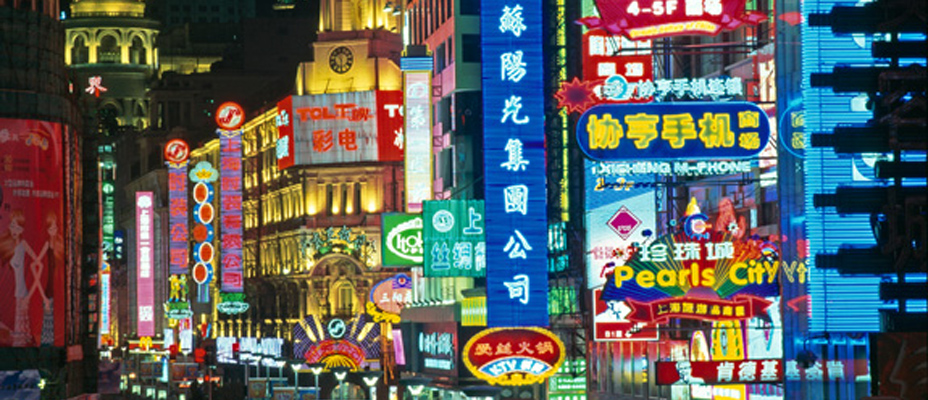
SHANGHAI CHINA
The Shanghai International Exhibition Center, an example of Soviet neoclassical architecture in Shanghai